Our Team
This is the group for the Total Knee Arthroplasty Gap Balancer With Calibrated Tensioning. All members are 4th year Biomedical Engineers.
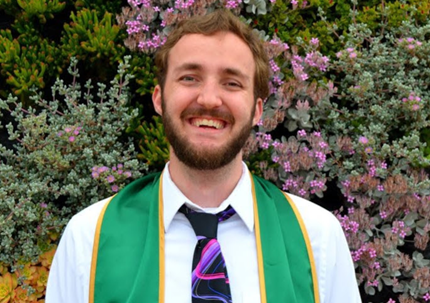
Corey Catuara
Conceptual Design and Testing Lead
Corey is a biomedical engineering major with a mechanical design concentration from Rancho Cucamonga CA. His main roles were to develop conceptual designs, create the manufacturing and assembly instructions, design and execute testing procedures for each specification, and develop changes to be made in future iterations of the device. He also aided in specification development, checked all detailed drawings, and determined appropriate part tolerances.
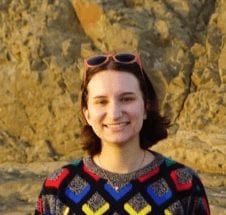
Lauren Heningman
Solid Modeling and Data analysis LEad
Lauren Henigman is a biomedical engineer from Santa Clarita, CA. Her main roles in this project were part and assembly modeling as well as testing data analysis. Lauren also assisted in part manufacturing and prototype assembly.
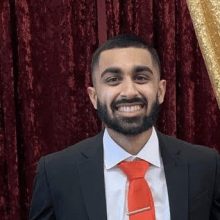
Jaypreet Dhanota
Manufacturing Lead
Jaypreet Dhanota is a biomedical engineer from San Jose, CA. His main roles were managing manufacturing and assisting in assembly. Jaypreet also helped with specification and design development and constructing the main apparatus for testing.
Acknowledgements
We would like to thank our sponsor, Dr. Delegrammaticas, and the machine shop techs at Cal Poly who helped us fund and create our prototype, respectively. Further, we would like to thank Cal Poly for providing us with the facilities to create our project.
Our Project's Video
Our Project's Digital Poster
Indications for Use
The total knee arthroplasty calibrated tensioner is indicated for use in conjunction with the corresponding gap balancer device in skeletally mature patients who qualify for a total knee arthroplasty. The product should be used by surgeons who have experience with the total knee arthroplasty procedure. The product can be used in a gap balanced technique for total knee replacement to measure the gap between the femur and tibia to ensure that appropriate cuts have been made, and to ensure that the medial collateral and lateral collateral ligaments are tensioned properly prior to the insertion of the replacement knee.
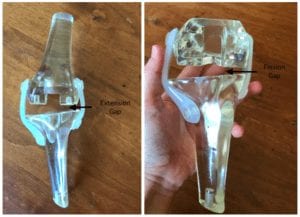
The main goal of the project was to create an instrument that coexists with the gap balancing devices created by a previous group to properly and consistently tension the soft tissue while the surgeon checks that the extension and flexion gaps are symmetric.
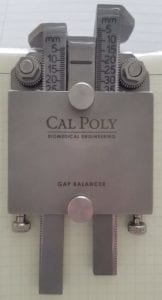
Specification Development
Through collaboration with our sponsor Dr. Delagrammaticas, we created a list of engineering specifications that we could use to evaluate the functionality of our device.
Specification | Description | Target |
Precision | Tension measurement consistency tolerance | ±0.5 lb |
Ease of Use | Mean ease of use score on a scale from 1 to 10 | 7 |
Force required by user to operate | 10 lb | |
Device works with both paddles | Yes | |
Previous design user interface visible | Yes | |
Material Properties | Material is biocompatible | Yes |
Temperature material can withstand | 121°C | |
Pressure material can withstand | 20 psi | |
Material doesn’t react with sterilization chemicals | Yes | |
Reliability | Device can withstand expected stresses | Yes |
Size | Maximum design size | 12x5x3 in |
Minimum design size | 3x2x1 in |
Design Process
The first step in the design process was to create a design concept based on different methods to move paddles, measure tension, and display tension. After creating a design morphology, we selected a method that we referred to as the slipper method. This method uses a non-ratcheted lever to apply torque at the hexes and move the paddles, and it combines measuring and displaying tension. In this design, a sliding base is used to alter the compression of a spring, which causes the spring to apply a force to a bullet, which applies an equivalent normal force to the edge of a CAM wheel. When the user uses the device to apply a torque, the friction force between the bullet and the wheel prevents the wheel from moving, letting the user turn the gap balancer hexes until the torque required to continue turning the hex exceeds the friction force between the bullet and CAM wheel, at which point the wheel will slip and rotate freely without turning the hex, preventing it from lifting the paddles any further.
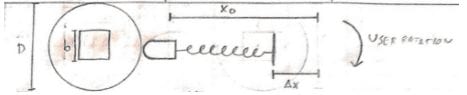
The next step in the design process was to determine the best shape for the CAM wheel to maximize the output torque of the device while minimizing required spring force and design complexity. To do so, we evaluated three different shapes for our CAM wheel: a star shape, a flower shape, and a simple circle. Our analysis showed that the circular CAM wheel would be the most efficient method from a manufacturing and complexity standpoint, as the more complex options did not add significant value to the design’s functionality or precision.
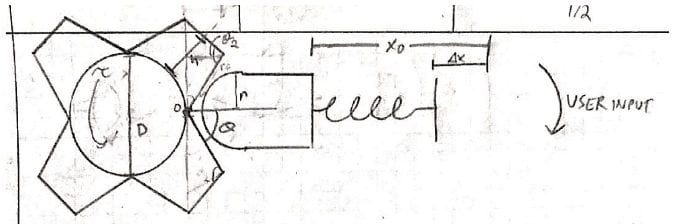
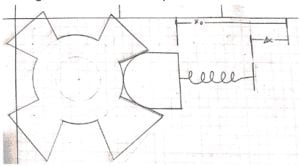
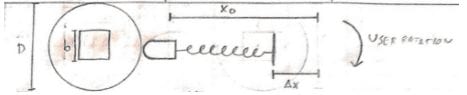
The last major step was to choose a mechanism for setting the base of the spring and locking it in place. We considered either using a ratcheting system that the user pushes or using a threaded bolt that the user turns to raise the spring base. After some deliberation, we determined that a knob which turns a threaded bolt would be simpler to manufacture, more intuitive within a surgical setting, and would require less force from the user to operate. Once these design decisions had been made, we created a detailed design modeling the parts and assembly using SolidWorks.
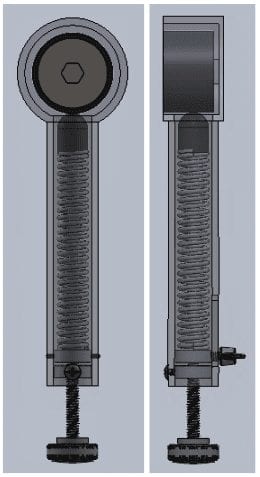
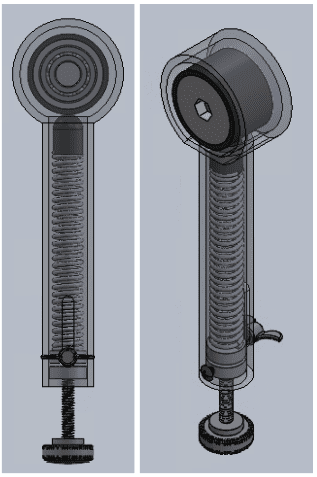
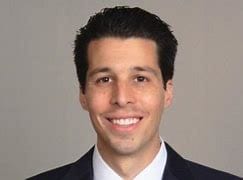
This project is sponsored by Dr. Dimitri Delagrammaticas, MD
Manufacturing
We began manufacturing by adhering the ball bearing to the wheel casing, then placing the rubber sleeve around the CAM wheel.
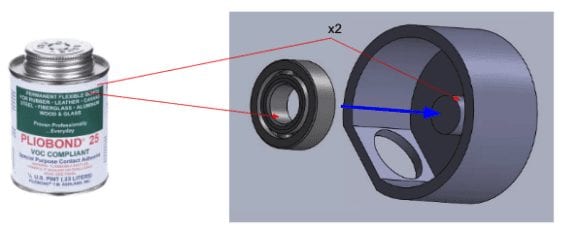
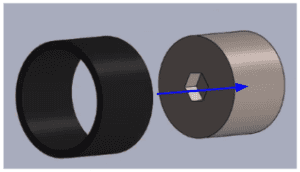
We continued by adhering the CAM wheel to the ball bearing and wheel casing. Next, we adhered the tube casing to the wheel casing.
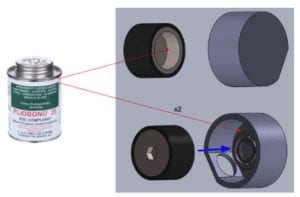
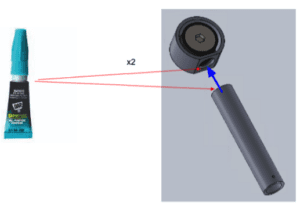
We then adhered the flat end of the bullet to the spring and the other end of the spring to the slider base.
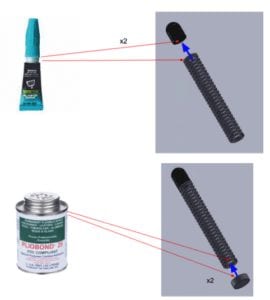
Next, we inserted the newly mated spring, bullet, and slider base into the tube casing such that the threaded hole in the slider base aligned with the slot in the tube casing. We then screwed the indicator screw into this threaded hole in the slider base.
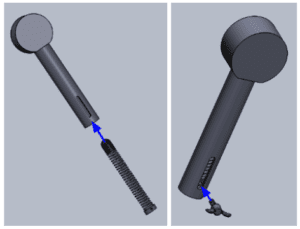
We then screwed the threaded end cap into place at the bottom of the tube casing. Finally, we inserted the threaded knob into the hole in the end cap to complete the assembly.
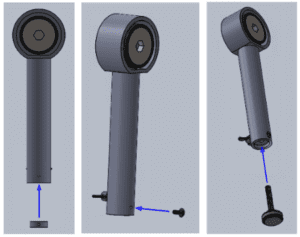
Testing for Precision
Our main test for functionality was a test for precision, which evaluated the tension measurement precision between measurements based on the level of tension and the paddle being used. The goal of this testing was to show that tension measurements maintained similar precision that was within the acceptable range of variation under all use conditions.
To facilitate this testing, we constructed a testing apparatus out of wood, using the dimensions specified in the figures below. We also purchased bungee cords, which we used to set the desired tension for each set of trials.
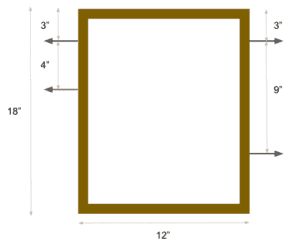
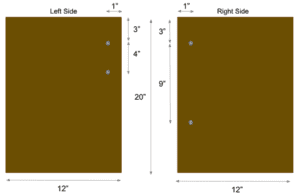
Before beginning testing, we placed the gap balancer on top of the testing apparatus on the side closest to the screws, and after centering it, we used duct tape to fasten it in place. Each test began by setting the bungee cords up in the configuration shown in the table below. In this table, T = top and B = bottom, where the first letter listed refers to where the bungee is hooked on the left side of the testing apparatus, and second letter refers to where the bungee cord is hooked on the right side of the testing apparatus.
Trial Number | Tension Setting (N) | Paddle | Bungee Configuration | ||
1 | 2 | 3 | |||
1 | 28 | Left | TT | TT | n/a |
2 | 35 | Left | BT | n/a | n/a |
3 | 35 | Right | BT | n/a | n/a |
4 | 64 | Right | BT | TT | TT |
5 | 72 | Left | TT | TB | n/a |
6 | 92 | Right | TB | BT | n/a |
7 | 109 | Left | BB | TT | TT |
8 | 116 | Right | TB | TB | n/a |
9 | 148 | Left | TB | TB | BT |
10 | 150 | Right | TB | TB | BT |
11 | 149 | Left | TB | TB | BT |
12 | 153 | Right | TB | TB | BT |
It is important to note that the tension setting value given for each set of trials were determined after testing based on the geometry of the bungee cords at the mean final displacement. This is the reason for the odd selection of tension values, and the small differences in the tension setting for the final 4 sets of trails allowed us to determine a design problem: the spring we chose was buckling and therefore wouldn’t displace the same way in the later trials as in the earlier ones.
To begin testing, we set the calibrated tensioner to the appropriate tension setting and used it to raise the appropriate paddle until no further extension of the paddle was observed. We recorded this maximum displacement reached by the gap balancer then reset the gap balancer paddle to its lowest position. We repeated this for a total of 8 measurements for each set of trials.
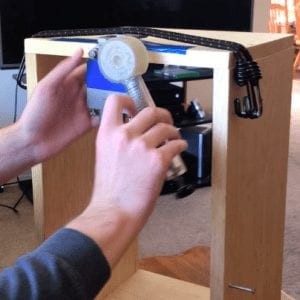
During testing, it became clear that at higher tension values, the CAM wheel sleeve was problematic, as it frequently would get pushed out of place, requiring the user to stop and put it back in place. We decided to do some additional testing without the CAM wheel sleeve to see if this was a viable approach, and this change lead to a significant increase in the device’s precision, as can be seen in the graph below.
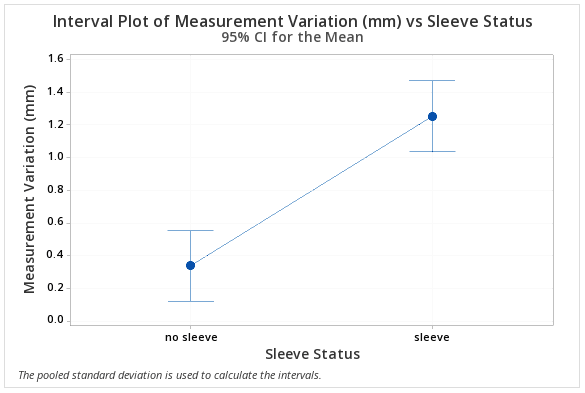
The results of the precision testing without the sleeve are shown below. Our goal was for the device to provide measurements that are precise to within 0.5 lbf (~2.2 N), and the results demonstrate that our device was compliant in all cases.
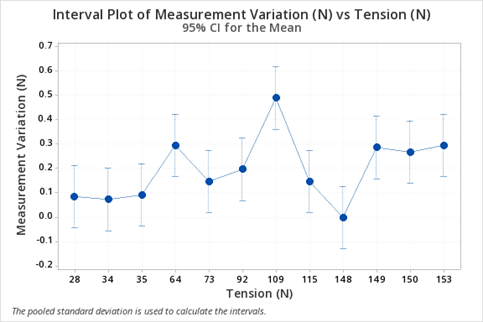
Final Design
Specifications
Dimensions: 8.7” X 2.25” X 1.25”
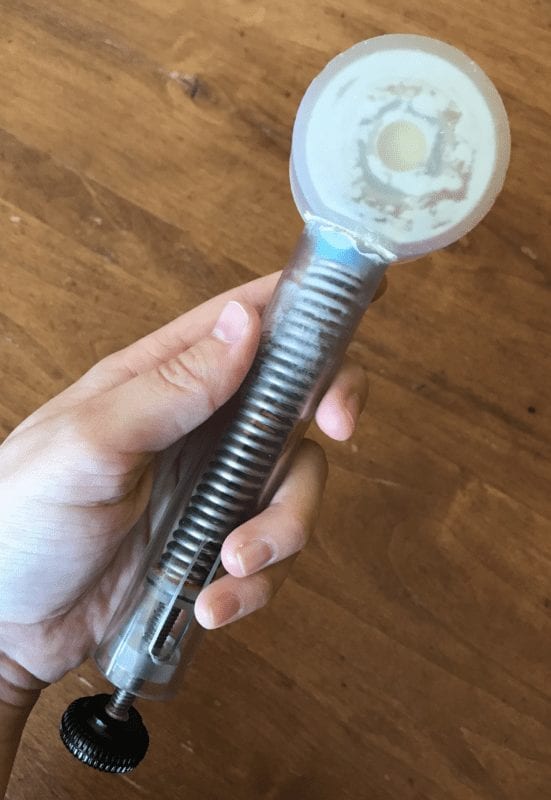
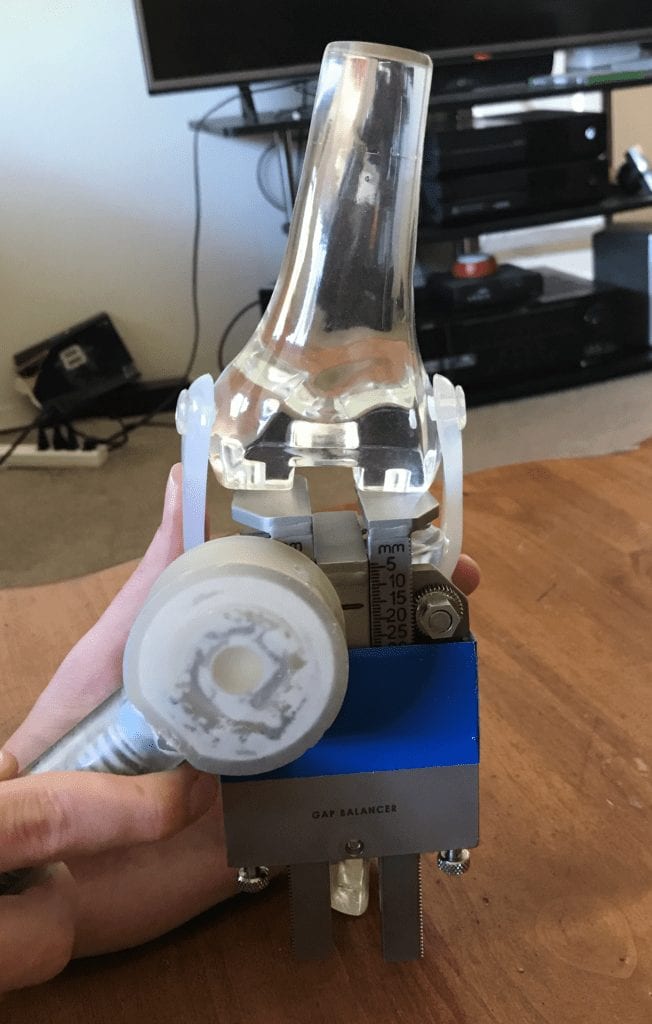
Custom Components
– CAM Wheel
– CAM Wheel Case
– Tube Casing
– Slider Base
– Threaded End
– Bullet
– Wheel Casing
Material
– High Temp SLA Resin
– High Temp SLA Resin
– Polycarbonate
– Polycarbonate
– Polycarbonate
– Silicone Rubber
– Silicone Rubber
Stock Components
– Ball Bearing
– Knob
– Screws
– Spring
Material
– Stainless Steel and PEEK Plastic
– Stainless Steel and Phenolic Plastic
– Stainless Steel
– Stainless Steel
Additional Testing and Results
Much of our testing was simply variations of the test for precision designed to isolate certain specifications to check if the device meets the requirements. For the user force test, a spring scale was used to turn the calibrated tensioner in the trials, and we recorded the force required at each level of tension. We then used a line of best fit to determine the output tension when the user was applying the maximum acceptable amount of force. A line of best fit had to be used to extrapolate since the spring scale did not go to a high enough force value to test directly. The results of this test can be seen in the graph below.
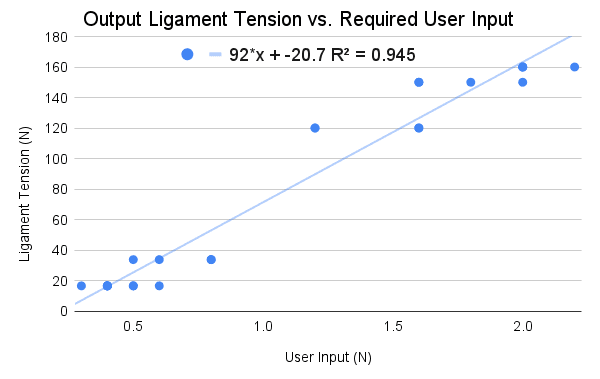
For the ease of use test, we had Dr. Delagrammaticas complete a few trials using the same procedure as the precision testing. After doing so, he gave the device an ease of use score on a scale from 1-10. He ended up rating the current iteration of the device as a 7, which met our requirement.
For material property testing, we completed a literature review of each material used in the device to ensure that it met the specifications of being both biocompatible and autoclave compatible. Due to issues in manufacturing, the selected adhesive could not be used to attach the tube casing to the CAM wheel housing, and superglue had to be used instead. Because this adhesive is not autoclave compatible or biocompatible, the final prototype failed to meet these specifications.
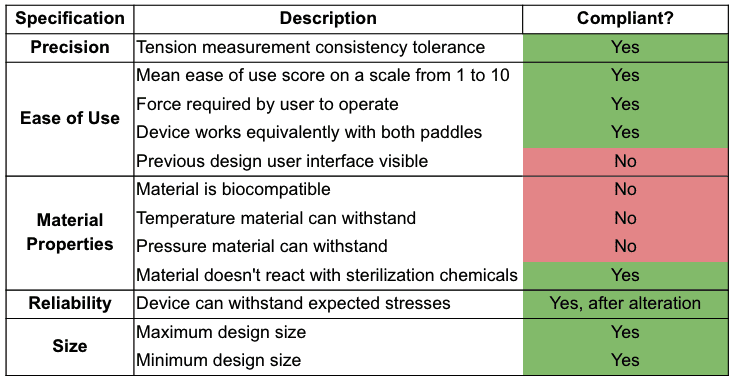
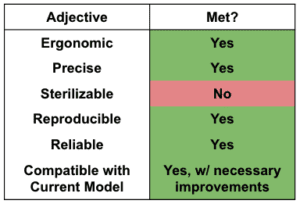
Our prototype succeeded in meeting the criteria for precision, most of the criteria for ease of use, and the criteria for size and reliability. Our prototype failed to meet the previous design user interface visibility criteria and the material property criteria, for reasons previously mentioned. While our prototype was unable to satisfy all customer requirements and specifications, we did manage to create a strong, valid proof-of-concept to support the overall validity of this device. With the specific recommendations that we have suggested, we expect that all requirements and specifications will be met in future iterations.
Future Iterations
For future iterations, we offer the following recommendations:
We suggest that the changes outlined in the table below are made to the dimensions of the appropriate parts.
Part | Previous Dimension | New Dimension |
CAM wheel outer diameter | 1.4” | 1.3” |
CAM wheel casing outer diameter | 2.25” | 1.625” |
CAM wheel casing thickness | 0.15” | 0.1” |
Distance from bottom of tube housing to lower centermark of slot | 0.51” | 0.41” |
Distance from bottom of tube housing to upper centermark of slot | 1.71” | Calculate using desired maximum tension according to x=d/(μ*k*D)*Flig+0.3″ |
Slider base thickness | 0.25” | 0.2” |
Threaded end cap thickness | 0.25” | 0.2” |
Spring | See note below |
We suggest that all parts made from polycarbonate, SLA, and silicone rubber are instead made out of 316L stainless steel. This will increase the device reliability and should eliminate the risk of galvanic corrosion from using different metals.
We suggest that the tube casing and the CAM wheel housing are welded together rather than attached using an adhesive. This will make assembly easier and will increase device reliability.
We suggest that the spring to be used in future iterations is made of 316L stainless steel and has the following parameters: an outer diameter of 0.825”, a wire diameter of 0.095”, a free length of 4”, a solid length of 2.75”, a spring coefficient of 24 lbf/in, 28 turns, and closed ends. This design will prevent buckling in conjunction with the inner diameter of the tube, as there is only 0.05” of diametral clearance between the spring and the wall.
We suggest that both the bullet and CAM wheel are cast out of 316L stainless steel and machined to the desired surface finish.
We suggest that the new coefficient of friction between the bullet and CAM wheel is found by casting a large block and a 0.6” diameter disc, placing the disc on top of the block, tilting the block until the disc slips, and analyzing with statics. Once this has been done, the distance from the bottom of the tube casing that corresponds with a specific output tension can be found according to the following formula: x=d/(μ*k*D)*Flig+0.3″, where d is the diameter of the pinion on the gap balancer, D is the CAM wheel diameter, k is the spring constant, and μ is the coefficient of friction.
We suggest that the indicator screw be removed and that the slider base be made into a solid disc, with the end farthest from the CAM wheel being used as the reference point for the scale. This is because in practice, the indicator screw hurt ease of use rather than helping.
We suggest that the spring, bullet, and slider base are welded together rather than using adhesive to increase reliability and make assembly easier.
We suggest welding the threaded end cap to the tube casing rather than attaching it using a screw to decrease device bulk and increase reliability.
Conclusion
Through the use of various conceptual models and decision making tools, we were able to determine the ideal configuration for our device. Most notably, this configuration included the use of what we refer to as the “slipper method” with a round CAM wheel and a knob adjustment locking system. Subsequently, we were able to determine if the customer requirements and resulting engineering specifications were met by our prototype and overall design through extensive testing. Our prototype succeeded in meeting the criteria for precision, most of the criteria for ease of use, and the criteria for size and reliability. Our prototype failed to meet the previous design user interface visibility criteria and the material property criteria. While our prototype was unable to satisfy all customer requirements and engineering specifications, we did manage to create a strong proof-of-concept to support the overall validity of this device and its chosen form. With the specific recommendations that we have suggested based on testing and experience, we expect that all requirements and specifications will be met in future iterations.